News Story
New Developments in Power Electronics Could Improve Sustainability Efforts
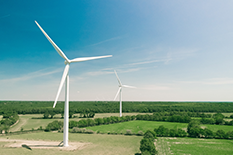
Power Electronics, and more specifically power electronics devices, will change the way we utilize and control renewable energy systems. A completed project, funded by the Navy and the Defense Threat Reduction Agency as well as the National Science Foundation, has developed a new class of power switching devices based on material systems, transforming performance to new levels of achievement and will ensure reliability and system robustness at the device level.
The Gallium Nitride (GaN) and the related ternaries, Aluminum Gallium Nitride (AlGaN) and Diamond, are the materials of choice due to their superior properties. While material and reliability issues limit the performance of the current generation of GaN devices, the promise remains an elusive goal. The project has resolved the issues of performance and reliability for GaN and related compound power devices. Also, it has developed a new class of devices based on Vertical Groove High Electron Mobility Transistors (V-HEMTs) and P-Type High Electron Mobility Transistors (p-HEMTs) to exceed the state of the art of 2.5 kV for Silicon and Silicon Carbide (SiC) devices for blocking voltages up to 10 kV and beyond. During 2020-2021, thick epi characterization and the understanding of defects in vertical GaN devices will lead to achieving breakdown voltages beyond 20 kV. The project has also developed reliability models which have shown that the power electronics module is the key component necessary to achieve reliable operation of the entire turbine over its projected 25-year life span.
The design, fabrication, and testing of structures exploiting the V-groove HEMT GaN structure combined with the trench Metal Oxide Semiconductor (MOSFET) concept have outperformed state-of-the-art Silicon and Silicon Carbide switches. Material-device-performance correlation methodology has ensured that the reliability is designed in, through the physics of failure approach. The project has also assessed other novel structures with blocking voltages over 10kV, as well as the evaluation of the reliability of these devices under system environments. The device has been the vertical enhancement-mode GaN-AlGaN HEMT, which has increased the operating frequency. These devices dramatically go beyond power MOSFETs and Induction Gate Field Effect Transistors (IGFETs) and will not require stacked, identical p-n junctions required for superjunctions.
The Impact
The major outcome of a reliable GaN technology and Wideband Gap Semiconductor device technologies for wind power turbines can be determined at the systems level by enabling reduced system size and cost, maximizing speed, high voltage handling capabilities, maximizing power output. The total life cycle system costs will be greatly reduced. Smart Power Modules based on active electronically scanned voltage switching will dominate all application areas in the energy sector. The performance enhancement possible through inserting the emerging wide band-gap semiconductor power devices can only be possible if all degradation mechanisms are understood and reliability can be achieved.
New Research Directions and New Industries
The GaN Vertical Heterojunction Field Effect Transistor (HFET) technology can have a significant impact on the next generation of power modules due to their one order of magnitude higher power density over conventional Silicon IGFETs, and their ability to operate at higher efficiency and voltage linearity and because of their robustness to incident power immunity in many grid applications. However, the reliability improvements necessary to achieve this promise requires the development of a new physics-based approach to reliability prediction for GaN-based High Voltage Field Effect Transistors (HVFETs). This has been accomplished through the development of reliability models based on the dynamic Bayesian approach.
Innovations and Interdisciplinary Character
This project has culminated in the development of physics-based failure models for GaN-based HVFETs which by itself is innovative. More importantly, this has established an integrated fundamental science approach encompassing degradation processes such as the material/heterostructures, bulk, surface and interface states, temperature, strain, and high field effects such as hot carriers and hot phonons generation as well the materials science of contacts and Schottky barriers. The point defect parameters and their effect on device reliability have been determined and include surface, interface, bulk barrier, and bulk buffer traps. The physics of hot phonons and hot electrons and their interplay with thermal and polarization phenomena as well as interactions with existing defects and the nucleation of new defects have been uncovered. The effect of hot electron and hot phonon on phonon decay and power dissipation, as well as the velocity limiting processes, has been determined. All of these factors, once eliminated, lead to system integration of High Voltage GaN Three Terminal Devices in reliable wind turbines.
An Upcoming Challenge
The establishment of the basis for the 21st-century approach to robust manufacturing and reliability has begun. To meet this challenge, the failure mechanisms due to the high electric fields, the coupled effects of heat, strain, and electric polarization, gate current, and the relatively high density of extended and point defects endemic in GaN and SiC must be further identified and quantified. For example, Professor Christou and students have identified unique dislocation clustering in thick GaN epitaxial layers necessary for power electronics. Such clustering must be controlled and understood from a thermodynamics point of view. Such clustering is shown below. The physics-based knowledge gained through extensive and targeted characterizations and analyses must be incorporated into the failure models which can then become the basis for the new robust manufacturing approach. This then could become the basis for the new methodology for reliability prediction and manufacturing science for future technologies and will directly impact energy systems and operations.
Offshore wind energy is one of the critical applications due to the harsh operating environment. This is of specific interest to the state of Maryland as a large “wind farm” will soon be operating in the Atlantic Ocean, approximately 20 miles from Ocean City, MD.
For more information on this research, contact Prof. Aris Christou.
Published February 7, 2020